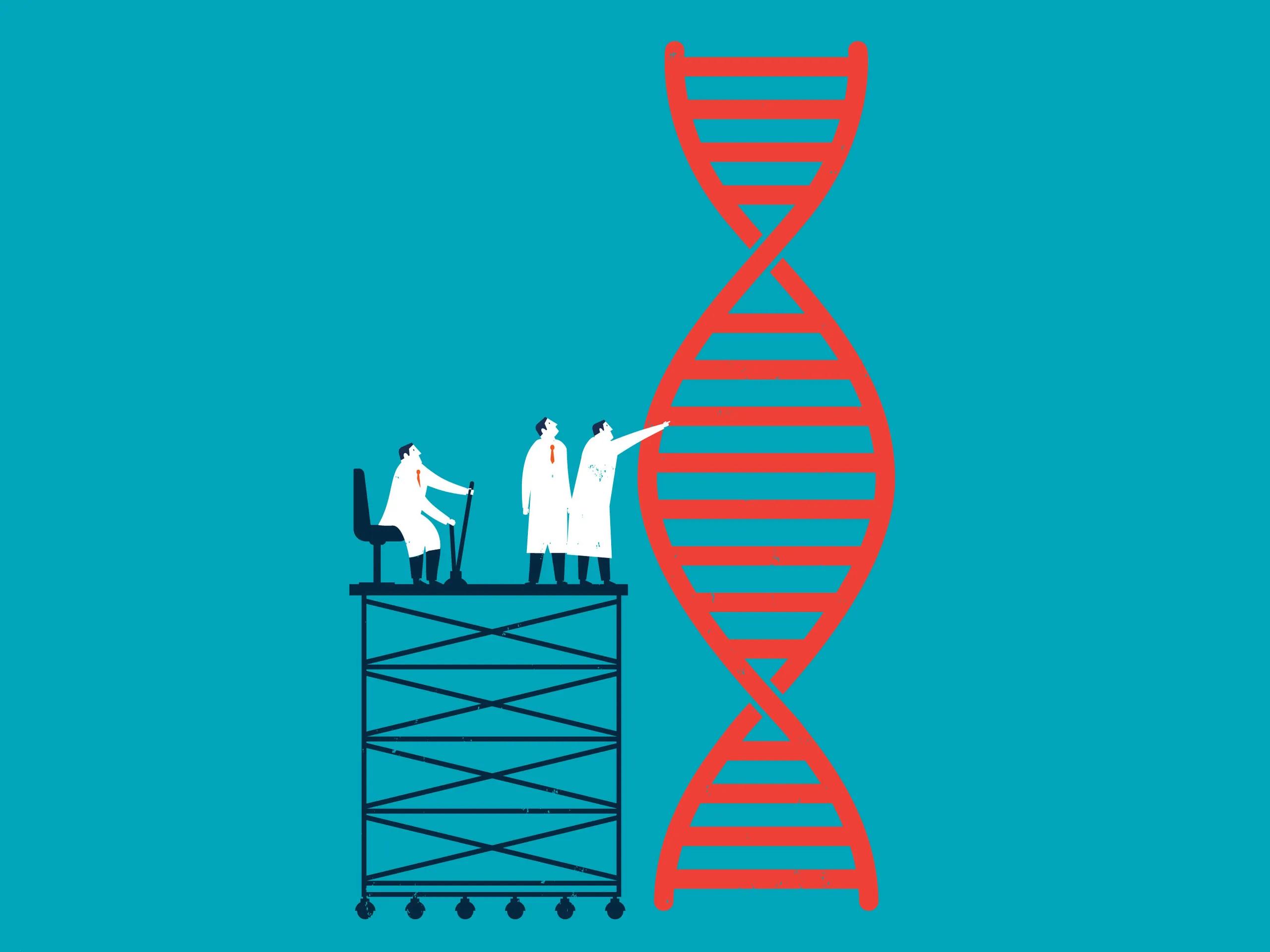
Gene mapping is a cornerstone of genetics, providing a detailed understanding of the locations of genes on chromosomes. This crucial technique aids researchers in uncovering the genetic underpinnings of traits, diseases, and biological processes. By exploring the arrangement and distances between genes, scientists can illuminate the complexities of inheritance, leading to significant advancements in medicine, agriculture, and evolutionary biology.
Two Main Approaches to Gene Mapping
Gene mapping can be categorized into two primary approaches: Types of Gene Mapping
Genetic Linkage Mapping and Physical Mapping.
Genetic Linkage Mapping: This method focuses on the inheritance patterns of genes. By examining traits that are passed down through generations—such as physical characteristics or disease susceptibility—scientists can construct linkage maps. These maps depict the relative positions of genes based on recombination frequencies observed during meiosis. This approach helps identify which genes are likely to be inherited together, revealing their proximity on the chromosome.
Physical Mapping: In contrast to linkage mapping, physical mapping provides precise measurements of the distances between genes, often quantified in base pairs. Techniques like radiation hybrid mapping and DNA sequencing are used to create accurate physical maps. By assembling overlapping DNA fragments, researchers can depict the actual layout of genes on chromosomes, offering a comprehensive view of genetic architecture.
Historical Context
The field of gene mapping was first advanced by Alfred H. Sturtevant in 1913 when he developed the first genetic map using Drosophila (fruit flies). By studying recombination frequencies, he was able to calculate distances between genes, laying the groundwork for modern genetic mapping techniques.
Techniques in Gene Mapping
Various techniques are utilized in gene mapping, each tailored to specific research applications:
Restriction Fragment Length Polymorphism (RFLP): This technique measures variations in DNA sequences to estimate distances between genetic markers, providing valuable insights into genetic diversity.
Single Nucleotide Polymorphisms (SNPs): Commonly used in Genome-Wide Association Studies (GWAS), SNPs are single-base variations that help identify genetic differences across populations.
Amplified Fragment Length Polymorphisms (AFLP): Effective for mapping complex genomes, AFLPs offer markers linked to traits of interest, aiding in both species identification and population genetics.
In cancer research, gene mapping is particularly vital. By pinpointing the locations of genes and mutations associated with different cancers, researchers can develop targeted therapies, improve diagnostic methods, and create personalized treatment plans, leading to better patient outcomes.
The Connection Between Gene Mapping and Genetic Testing
Gene mapping and genetic testing are interrelated fields that enhance our understanding of genetic disorders. Gene mapping identifies the locations of genes, while genetic testing utilizes this information to detect mutations linked to diseases. Together, these tools facilitate personalized medicine, enabling early diagnosis and tailored treatments based on an individual’s unique genetic profile.
Conclusion
In conclusion, gene mapping is a powerful tool that has transformed our understanding of genetics and its implications for health and disease. By elucidating the genetic basis of traits and conditions, gene mapping contributes to the development of personalized medicine and targeted therapies. As technology continues to evolve, the integration of gene mapping into healthcare is expected to enhance diagnostic and treatment strategies, ultimately improving patient outcomes and deepening our understanding of the human genome.
Through the insights gained from gene mapping, researchers and clinicians are better equipped to tackle genetic disorders, offering hope for more effective therapies and an improved quality of life for those affected.
Write a comment ...